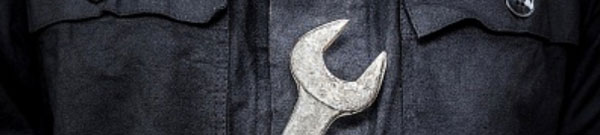
Electromagnetic Induction
An electrical conductor that can be moved freely is placed between the N and S (magnetic) poles of a magnet as shown in the diagram. Then, a galvanometer is connected to the conductor to complete a circuit.
When the conductor is moved between the magnetic poles as shown in the diagram, the indicator of the galvanometer swings.
Thus, when the conductor is moved between the magnetic poles, the conductor crosses and cuts off the magnetic flux, which generates a current. For this reason, if the conductor is moved parallel to the magnetic flux, no current will be generated.
This phenomenon that generates current is called an electromagnetic induction, and the current that flows through the conductor is called an induction current.
The induction current is generated by the electromotive force that is created in the conductor as a result of the electromagnetic induction. This electromotive force is therefore called an inductive electromotive force.
Direction of Electromotive Force
the relationship between the direction of the magnetic field, the direction of the inductive electromotive force, and the direction in which the conductor is moved. This relationship is generally known as Fleming’s right-hand rule.
According to this rule, the following applies when the thumb, index finger, and middle finger of the right hand are opened to form right angles:
Index finger: Direction of flux (B)
Middle finger: Direction of current (I)
Thumb: Direction of motion (F).
Amount of Electromotive Force
A conductor that moves at a constant speed in one direction, between magnetic flux lines having the same density
A conductor that moves at a constant speed in the path of a circle between magnetic flux lines having the same density
The amount of the inductive electromotive force is directly proportionate to the number of magnetic flux lines that the conductor disrupts per unit of time.
The inductive electromotive force of a conductor, which moves at a constant speed in one direction between magnetic flux lines of the same density, is the same at any point.
However, if the movement direction of the conductor is not the same, the electromotive force will vary even if the speed is constant and the magnetic flux is of the same density.
In the diagram, the conductor rotates counterclockwise around point 0, between the magnetic poles.
When the conductor is in positions 0 and 6, the direction of the magnetic flux and the movement direction of the conductor are parallel to each other. Therefore, it will not generate an electromotive force.
Conversely, when the conductor is in positions 3 and 9, the direction of the movement of the conductor crosses the magnetic flux perpendicularly. This creates the greatest amount of electromotive force.
The sine graph below represents the relationship between the movement direction of the conductor and the amount of electromotive force.
Principle of the Generator
When a single conductor rotates in a magnetic field as shown in the diagram, an inductive electromagnetic force will be generated through electromagnetic induction.
When the conductor is bent and rotated as shown in the diagram, twice the amount of inductive electromotive force will be generated.
When the conductor is formed into a coil as shown in the diagram, greater amount of inductive electromotive force will be generated. In this manner, the rotation of the conductor in the magnetic field generates an inductive electromotive force.
The greater the number of windings in the conductor, the greater the amount of inductive electromotive force that will be generated.
Alternating-current Generator
The amount and direction of the inductive electromotive force that is generated by the rotation of a coil will vary depending on the position of the coil.
In diagram (1) on the left, the current flows from brush A to the light bulb. In diagram (2), the supply of current stops. In diagram (3), the current flows from brush B to the light bulb.
Accordingly, the current that is generated by this device is an alternating current. Therefore, this device is called an alternating current generator.
Self-induction Effect
When the switch in diagram is closed or opened, the magnetic flux in the coil changes. To create the same conditions without allowing the current to flow through the coil, it will become the same as moving a magnet in and out of the coil as shown in diagram .
Moving a magnet in and out of a coil causes an electromotive force to be generated in the coil. This electromotive force is generated regardless of whether a current flows in the coil.
Thus, the magnetic flux variances that result from the flow or stoppage of current through the coil cause the same coil to generate an electromotive force.
This phenomenon is called the self-induction effect.
Mutual Induction Effect
Two coils are arranged in the diagram. When the current that flows through one coil (primary coil) is changed, an electromotive force will be generated in the other coil (secondary coil), in the direction that prevents the magnetic flux in the primary coil from changing. This phenomenon is called the mutual induction effect.
A voltage transformer utilizes this effect. A voltage transformer, which is contained in the ignition coil of a vehicle, is used to apply a high voltage to the spark plugs.
Because the magnetic flux does not change if a constant current flows through the primary coil, no electromotive force will be generated in the secondary coil.
When the primary current is disrupted by turning the switch from ON to OFF, the magnetic flux that was generated by the primary current up to that point disappears suddenly. Thus, an electromotive force will be created in the secondary coil in the direction that will prevent the elimination of the magnetic flux.
Thus, a voltage transformer allows current to flow to the primary coil, and when the current is cut off, the high voltage that is generated by the self-induction effect of the primary coil is further increased between the primary and secondary coils through the mutual induction effect.
The amount of inductive electromotive force that is generated by this device changes with the following conditions:
The changing speed of the magnetic flux:
With a given amount of change in the magnetic flux, a change that occurs within a shorter time generates a greater amount of electromotive force.
The amount of magnetic flux:
The greater the amount of change of magnetic flux, the greater the electromotive force will be.
The number of windings of the secondary coil: With a given amount of change in magnetic flux, the greater the number of windings, the greater the amount of electromotive force will be.
Thus, in order to generate a high secondary voltage, the current that flows to the primary coil should be as great as possible, and then the current should be cut off suddenly.